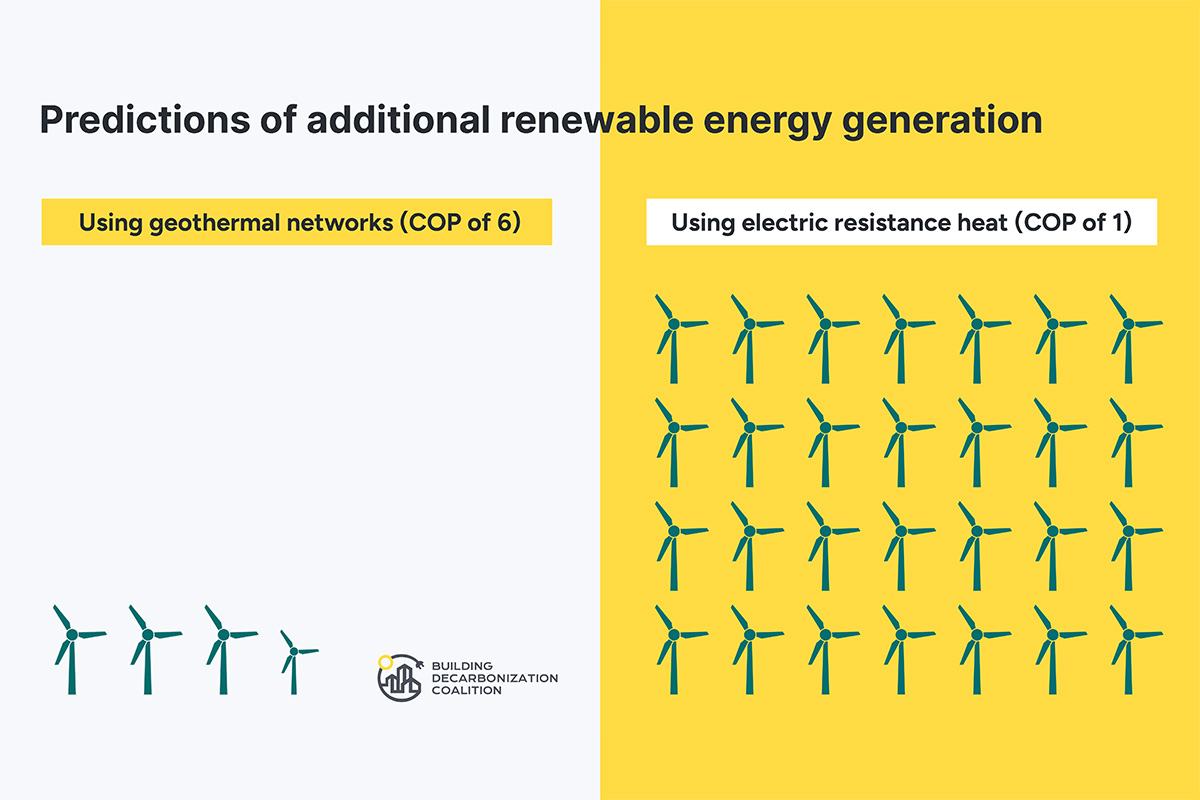
Affordable Heat, Efficient Grid:
Using Thermal Energy to Save Time, Money, and Energy
Efficient decarbonization could save the U.S. $557 billion in infrastructure costs.
The United States is on the cusp of a grand effort to electrify everything: to remove fuel combustion from industry, transportation, and buildings.1 This will require trillions of dollars and years of work.
So, like any audacious undertaking, we want to do it efficiently. In practical terms, we want to build resilient and durable decarbonized systems economically without burdening ratepayers. And we want to do this at a smart pace, without straining communities or the electric grid that serves them.
There are a multitude of approaches and technologies that will help us do this. We need to use them all: from reducing energy consumption in buildings, to monitoring electricity usage with smart meters, to installing energy-efficient appliances in homes and offices.
And when it comes to space heating and cooling, you may have heard that heat pumps are already more efficient than a gas furnace or an air conditioner. You may have even heard that “thermal energy networks are the most efficient system known for delivering heating and cooling.” (If that sounds familiar, thank you for reading our Thermal Energy Networks webpage.) But the full impact of these claims isn’t always obvious.
It matters because efficiency has huge consequences for electrifying affordably and wisely. Here, we’ll take the term “efficiency” out of the realm of buzzwords and ground it in a metric called the coefficient of performance, or COP. We will explain how this single-digit metric can, according to some calculations, translate into 12-digit savings: hundreds of billions of dollars.
We’ll explain how highly-efficient energy systems—heat pumps in general, and in this case, geothermal networks in particular—translate to saving precious time, money, and effort as we electrify.
Get to Know the Coefficient of Performance (COP)
We measure the efficiency of electric heating and cooling systems by their coefficient of performance (COP). A system’s COP has implications for affordability at the household level and on a national scale.
The formula for COP is simple. It compares an appliance or system’s energy input to its heating or cooling output. If an electric resistance heater has a COP of 1, that means 100% of the electricity it uses produces an equivalent amount of heat. If a heat pump has a COP of 3, it means that 1 unit of energy provides 3 units of heat or cooling: it is 300% efficient.
(An appliance claiming “higher than 100% efficiency” may sound too good to be true, but it’s real. It’s possible because heat pumps do not generate heat from scratch, the way a furnace does. Instead, they use electricity to power compressors and fans that move large amounts of existing thermal energy from place to place with relatively little effort.)
In simplest terms, the higher the COP, the more efficient the system. In practice, a system doesn’t have a single, fixed COP. An appliance becomes less efficient when there is a big difference between the ambient (outdoor) temperature and the desired indoor temperature. Figure 1 compares common average COPs (or equivalent) for different heating and cooling appliances or systems.
Figure 1: Comparison of COP (or equivalent) for common household heating systems. Sources: ENERGY STAR® Program Requirements Product Specification for Furnaces (April 2024); Winkler & Ramaraj (2023); Liu et al. (2023); XCel Energy2 / Buonocore et al. (2022)
On the far left is the gas furnace. The standard efficiency rating for furnaces is actually not COP—it is the Annual Fuel Utilization Efficiency, or AFUE, which measures the conversion of fuel into heat. But if we were to compare, a high-efficiency Energy Star furnace might convert 97% of its fuel into heat, so you could think of its COP as 0.97.3 This is generous: current Department of Energy manufacturing standards require furnaces to have a minimum AFUE of 80%, equivalent to a COP of 0.8. That minimum will increase in 2028.4 (Combustion can never achieve 100% efficiency).
Electric resistance heaters are slightly more efficient than gas furnaces, with a COP of essentially 1.5
The COPs of air-source heat pumps (ASHPs) vary depending on the exact unit and the outdoor air temperature. But based on field-tested studies of multiple ASHPs, a COP of 2.5 is a reliable average.6 This is where heat pumps begin to really show their superiority: they can heat, they can cool, and they are more than twice as efficient as the best gas furnace.
Geothermal or ground-source heat pumps (GSHPs) are even more efficient, with average COPs around 4.7 These heat pumps benefit from the relatively mild temperature just underground, which is often more stable than the air temperature; the appliances don’t have to work very hard to convert mild thermal energy into the desired temperature, even when the air outside is sizzling or freezing.
But the highest-known COPs come from networked GSHPs, also known as thermal energy networks (TENs) and specifically geothermal networks (GENs). The average COP of geothermal networks is an astonishing 6.8 That’s six times more efficient than the best gas furnace.
Key concept: What is thermal energy?
Thermal energy is the energy of the movement of molecules and atoms in all matter. It is everywhere, it is renewable, and it is measured in temperature: fast-moving particles are warmer than slow-moving particles. In theory, thermal energy only ceases to exist at absolute zero (-459.67°F or -273.15°C), the temperature in which particle motion would stop entirely. Otherwise, everything from snow, to air, to the ground beneath your feet contains thermal energy.
Thermal energy is associated with heat, but the two concepts are not exactly the same. Heat happens when fast-moving particles flow toward slow-moving particles. We physically experience that flow as heating or cooling. When you clasp a hot mug of tea, your hands feel heat as thermal energy flows from the liquid to your skin. When you clasp a frosty beverage, the sensation reverses.
This is the same mechanism that heat pumps use to cool and heat buildings. In cooling mode, heat pumps pull thermal energy from one place and push it somewhere else. That’s what your refrigerator does every day. In heating mode, heat pumps pull thermal energy from the air, ground, or water, and convey it within a building that needs it.
WATCH: “What is thermal energy?” video READ: The Switch Is On: How heat pumps work
How geothermal networks gain efficiency
Thermal energy networks—an umbrella term for a family of heating and cooling systems that includes geothermal networks—typically comprise multiple ground-source heat pumps (GSHPs), in separate buildings, all connected by an underground loop of pipe. When water circulates through that pipe, it becomes a thermal energy highway. Water whisks heat away from places that must stay cool—like data centers, ice arenas, or grocery stores—and delivers it to places that need warm air or water. In each building, the GSHP acts as a gatekeeper, rejecting or accepting heat. This trade of heat minimizes waste and boosts efficiency.
Geothermal networks, in particular, achieve high COPs thanks to two crucial design components: boreholes and an ambient-temperature loop (ATL) of pipe.9
Arrays of boreholes in a geothermal network typically burrow into soil or bedrock. The water-filled pipe loops through the boreholes, and the earth hugs that pipe, absorbing its thermal energy like a sponge and acting as a “battery” to store heat with very little dissipation.10
The underground temperature is consistently a mild ~55°F in most parts of the country.11 The water flowing through the pipe equalizes its temperature to match that of the ground. By the time this mildly-warmed water reaches a building’s GSHP, minimal electricity is needed to raise or lower its temperature. This consistent performance addresses two efficiency challenges simultaneously: it means geothermal networks can maintain high efficiency across seasons, and it reduces demand on the electrical grid during peak periods (particularly in winter, when heating needs are highest).
Data from Colorado Mesa University bears out this efficiency: its geothermal network can achieve an impressive heating COP of 8.9 in the winter, and 5.7 overall.
Figure 2: Components of a geothermal network. GSHPs are represented by the purple boxes on the sides of the buildings.
How efficiency helps you save money
It seems logical that a more-efficient system would save money compared to a less-efficient system. But the math does not always pencil out that way.
In all states, the average unit price of methane (“natural”) gas—measured in dollars per kBtu, or thousand British thermal units—is cheaper than the equivalent unit price of electricity.12 13 So, absent efficiency improvements and utility rate design that does not penalize electric appliances, electric heating and cooling systems are at a cost disadvantage.
But electric appliances do have a secret weapon: As described above, they are far more efficient than fossil-fueled appliances, and can leverage their efficiency to overcome this disadvantage. This is where the difference between a COP of 1, 2, and 6 starts to really matter.
Figure 2 compares utility rates for gas and electricity across the country to determine when heating with a heat pump is more cost-effective than a gas furnace. For example, if a household installs an air-source heat pump (ASHP) with an average COP of 2.5, there is an affordability tipping point if gas costs less than $1.20 per therm and electricity costs more than $0.13 per kilowatt-hour on average.
And whenever average electricity costs more than $0.11 per kilowatt-hour—which is true in the residential sector in 100% of the country14 —gas will be a more competitive price at $1.20 per therm.
But geothermal networks, with their COP of 6, are so incredibly efficient that they change this equation, even when the price of electricity is stacked against them.
Figure 3: Depending on your average utility rates, when does heating with a heat pump beat the cost of heating with a gas furnace? Source: U.S. Energy Information Administration
Colorado Mesa University’s ultra-efficient geothermal network is an example of these economic benefits. Without the energy-cost savings from geothermal heating and cooling, the school’s tuition would be 2% higher today.15
From the household to the nation
Now let’s expand our thinking from the scale of an individual system to the scale of an entire country.
In 2023, about 60% of the electricity in the United States was generated by fossil fuels like gas or coal.16 But renewable sources—such as wind and solar—are increasingly powering our electric grid.17
Simultaneously, building decarbonization and electrification are gaining momentum. One hundred and thirty-five municipalities and five states have passed building decarbonization ordinances.18 Heat pumps outsold gas furnaces for the second year in a row in 2023, and sales are strong in 2024.19 And recent federal legislation—the Infrastructure Investment and Jobs Act, the Inflation Reduction Act, and the CHIPs and Science Act—will release funding that, by one estimate, could double our current pace of building decarbonization.20
This electrification acceleration is exciting. But without complementary policies and technical innovation, a rapid, uncoordinated adoption of electric technologies has the potential to push our electric grid beyond what it can handle. We must build more, and new, forms of renewable power generation. We must also build new, and more, delivery infrastructure, like transmission lines and substations. In the words of Jesse D. Jenkins, assistant professor and energy systems engineer at Princeton University, “Most people alive today haven’t experienced the kind of building boom we must embark upon.” 21
This expected building boom will require thousands of skilled workers and trillions of dollars in a shrinking window of time. Decarbonizing efficiently is, then, an economic and policy imperative.
From the ground to the grid
The Department of Energy (DOE) has identified ground-source heat pumps (GSHPs) as a crucial decarbonization and cost-savings technology, even when they are not networked. The DOE’s Oak Ridge National Laboratory and the National Renewable Energy Laboratory published a study examining the potential outcomes of retrofitting 68%22 of U.S. buildings with non-networked GSHPs.23 (68% was the percentage of existing buildings the study authors deemed eligible for GHSP retrofits). It then modeled the subsequent costs of infrastructure buildout if the U.S. powered the entire electric grid with clean energy by 2050, consistent with federal climate goals.
A key conclusion: mass installation of GSHPs could avoid the need for up to 24,500 miles of new grid transmission lines by 2050. That’s enough to span the continental United States eight times. Avoiding this buildout would save $557 billion. 24
(Further, the study says, installing GSHPs also reduces the cost of electricity production and delivery and lowers the marginal cost of electricity by decreasing overall demand—resulting in lower energy bills. The study estimates cumulative savings of over $1 trillion by 2050 in present dollar value.)
“A key conclusion: mass installation of ground-source heat pumps could avoid the need for up to 24,500 miles of new grid transmission lines by 2050. That’s enough to span the continental United States eight times. Avoiding this buildout would save $557 billion”
Not only are these savings staggering, they could potentially go further. This study investigated non-networked GSHPs, assuming these appliances had a standard heating COP of 4 and a cooling COP of 6.5. Geothermal networks, with their average COP of 6, could further reduce the total electric capacity the modernized grid needs to deliver, decreasing the cost of system improvements.
That is what a 2022 study conducted by the Home Energy Efficiency Team (HEET), Harvard University, and Boston University investigated.
The study first identified peak electricity demand, then did the math to plan a grid that can meet that peak.25 Nationwide, buildings’ electricity demand is currently highest in the summer, when air conditioners draw mightily from the grid. But overall energy demand is highest in January, when the majority of buildings (around 62%) consume fuels such as gas, heating oil, or propane to stay warm.26 27
This study found that if all U.S. buildings currently burning fuels for heat converted to electric resistance heating, which has a COP of 1, then electricity demand in January would increase from 338 terawatt-hours (TWh) to 872 TWh, a 2.5-fold increase.28
To meet that demand, we would need a tremendous 28-fold increase in January wind generation or a 303-fold increase in January solar energy generation — plus long-term storage solutions for electricity generated in other months.
The study finds that using air-source heat pumps (ASHPs) with a COP of 2 considerably reduces these requirements, but still generates a high peak demand, and consequently requires significant buildout.
But if we decarbonize some of those buildings using geothermal networks, with their average COP of 6, the buildout massively shrinks.29 We still need to roll up our sleeves, but we only need to increase current wind generation 4.5-fold, or solar generation 36-fold, to serve the peak needs of an all-electric country.
Figure 4: A comparison of new wind infrastructure we would need if we decarbonize with geothermal networks (GENs) (COP of 6) vs. electric resistance heat (COP of 1). Source: Buonocore et al. (2022)
This is a more achievable target. Wise use of geothermal networks—and widespread installation of air-source heat pumps in the places where geothermal networks are not feasible—means less time needed to meet climate goals and less money spent upgrading the grid.
The billions of dollars needed for infrastructure buildout may seem abstract compared to your own building’s very tangible monthly energy bills. But these costs are closely related, because you—like all electric utility customers—pay for infrastructure investments on your utility bills.30
On top of electric grid spending, building occupants that use methane gas already pay for gas pipeline investments: a separate set of infrastructure costs for an entirely separate form of energy. And despite decarbonization trends and climate goals, utility spending on new gas infrastructure has tripled in the past decade.31 Customers will pay for these investments for years to come, long after many neighborhoods have electrified and no longer need that infrastructure.
Putting efficiency to work
We know that building and maintaining two competing infrastructure systems for heating and cooling is not efficient. And we know that geothermal networks, with their high COPs, are the most efficient heating and cooling systems available.
But the most efficient systems in the world are not useful if they are not actually used. So how do we get geothermal networks out of discussions and into the ground where it makes the most sense?
Utilities—whether investor-owned, cooperative, or municipal—are one possible answer. While there are several geothermal networks already in operation around the country (that’s how we know so much about their COPs), the majority are owned by universities or other private entities for the exclusive use of campuses or developments. More than any other institution, utilities have the power and responsibility to put efficient and affordable energy into the hands of the customers that depend on them. These institutions can also provide project management, a trained workforce, and access to capital financing to kickstart the process. All are key ingredients for neighborhood-scale decarbonization projects.
Several gas utilities have noticed geothermal networks’ promise in affordably adapting to a clean-energy economy. There are 20 utility-owned pilot programs in various stages of scoping, construction, or operation across the country. 32 But scaling this transition equitably requires further action.33
Local governments are crucial to identifying opportunities, organizing communities, and indicating interest in bringing geothermal networks to their residents. In Massachusetts, HEET awarded grants of up to $50,000 to municipalities that expressed interest in conducting geothermal network feasibility studies, and ensured that nearly 50% of the funding went to environmental justice communities.34 In Vermont, advocates, local leaders, energy commission members, and regional planners created a step-by-step “how-to” resource to help municipal leaders bring non-emitting thermal energy infrastructure to their towns, with or without utility involvement.35 Local elected officials and staff have access to town plans to add or improve existing infrastructure, advance residential or commercial development, or otherwise coordinate geothermal network installation with complementary city goals.
State legislatures have a key role in cultivating a legal landscape that’s conducive to heating and cooling with thermal energy. Lessons from early legislation and pilots have guided states toward legal provisions supporting environmental justice communities and organized labor—New York’s groundbreaking Utility Thermal Energy Networks and Jobs Act passed unanimously in 2022 thanks to the support of a coalition of utilities, advocates, and labor.36 As of September 2024, eight states have passed legislation mandating or allowing regulated utilities to develop thermal energy network pilots. Two states—California and Washington—have even reexamined the legal statute known as the “obligation to serve,” a common requirement that can prevent neighborhood-scale decarbonization, including with geothermal networks, if just one customer in a community prefers to use gas.37 Amending this statute can bring consumers more choice in their energy, and unlock existing funding to decarbonize with greater efficiency and long-term cost savings.
Conclusion
The infrastructure we build today will shape our energy landscape, and customers’ energy bills, for decades to come. Heat pump technology can be distributed thoughtfully to improve efficiency overall. Any heat pump will boast a higher COP than a gas furnace, and geothermal networks achieve the highest COP of all the systems we know of, squeezing the most benefit out of every unit of energy and potentially translating to real-world savings for anyone who pays an energy bill. Their superior efficiency over gas furnaces can make them cost-effective even when electricity rates are high.
Inevitably, thermal energy networks and geothermal networks will not be equally feasible in every location. Due to geological profiles, engineering obstacles, or other barriers, air-source heat pumps may be a better solution in some neighborhoods. It’s all the more reason to take advantage of this efficiency wherever we can. By wisely deploying geothermal networks alongside ASHPs, we have a shot at meeting peak energy demands while minimizing grid expansion and saving infrastructure costs to the tune of $557 billion.
Whether we are talking about a single system’s COP or the mind-bending figures that accompany national infrastructure spending, for many communities, this is a solution that may represent the right blend of sustainability and affordability. We have the data, and we know the scope of the task ahead. Now we need coordinated, multi-stakeholder action that can help us turn knowledge into operational, affordable, and sustainable systems for all.
[1] Plumer, Brad. “How Electrifying Everything Became a Key Climate Solution.” The New York Times, April 14, 2023. https://www.nytimes.com/interactive/2023/04/14/climate/electric-car-heater-everything.html
[2] Xcel Energy. Evaluating a Community Ground Source Heat Pump System at Colorado Mesa University. 2022
[3] ENERGY STAR®. “ENERGY STAR® Program Requirements Product Specification for Furnaces, Draft 1, Version 5.0.” ENERGY STAR. n.d. https://www.energystar.gov/sites/default/files/2024-04/ENERGY%20STAR%20Version%205.0%20Furnaces%20Draft%201%20Specification%20.pdf.
[4] Liu, Xiaobing, Jonathan Ho, Jeff Winick, Sean Porse, Jamie Lian, Xiaofei Wang, Weijia Liu, Mini Malhotra, Yanfei Li, and Jyothis Anand. Grid Cost and Total Emissions Reductions Through Mass Deployment of Geothermal Heat Pumps for Building Heating and Cooling Electrification in the United States. No. ORNL/TM-2023/2966. Oak Ridge National Laboratory (ORNL), Oak Ridge, TN (United States), 2023. https://www.ecfr.gov/current/title-10/part-430/section-430.32#p-430.32(e)(1)(ii).
[5] United States Department of Energy. “Electric Resistance Heating.” Energy Saver. Accessed September 9, 2024. https://www.energy.gov/energysaver/electric-resistance-heating.
[6] Winkler, Jon, and Sugi Ramaraj. “Field Validation of Air-Source Heat Pumps for Cold Climates.” National Renewable Energy Laboratory, 2023. https://www.nrel.gov/docs/fy23osti/84745.pdf.
[7] Liu, Xiaobing, Jonathan Ho, Jeff Winick, Sean Porse, Jamie Lian, Xiaofei Wang, Weijia Liu, Mini Malhotra, Yanfei Li, and Jyothis Anand. Grid Cost and Total Emissions Reductions Through Mass Deployment of Geothermal Heat Pumps for Building Heating and Cooling Electrification in the United States. No. ORNL/TM-2023/2966. Oak Ridge National Laboratory (ORNL), Oak Ridge, TN (United States), 2023. https://www.ecfr.gov/current/title-10/part-430/section-430.32#p-430.32(e)(1)(ii).
[8] Liu, Xiaobing, Jonathan Ho, Jeff Winick, Sean Porse, Jamie Lian, Xiaofei Wang, Weijia Liu, Mini Malhotra, Yanfei Li, and Jyothis Anand. Grid Cost and Total Emissions Reductions Through Mass Deployment of Geothermal Heat Pumps for Building Heating and Cooling Electrification in the United States. No. ORNL/TM-2023/2966. Oak Ridge National Laboratory (ORNL), Oak Ridge, TN (United States), 2023. https://www.ecfr.gov/current/title-10/part-430/section-430.32#p-430.32(e)(1)(ii).
[9] HEET and BuroHappold Engineering. “Geothermal Networks: 2019 Feasibility Study.” 2019. https://uploads-ssl.webflow.com/649aeb5aaa8188e00cea66bb/656f8ad67bbc7df081e3fe17_Buro-Happold-Geothermal-Network-Feasibility-Study.pdf.
[10] Skarphagen, Helge, David Banks, Bjørn S. Frengstad, and Harald Gether. “Design Considerations for Borehole Thermal Energy Storage (BTES): A Review with Emphasis on Convective Heat Transfer.” Geofluids, 2019.
[11] Kalogirou, Soteris, and Georgios A. Florides. “Measurements of Ground Temperature at Various Depths.” 2004.
[12] U.S. Energy Information Administration. “Electricity Data Browser.” Accessed September 17, 2024. https://www.eia.gov/electricity/data/browser/#/topic/7?agg=0,1&geo=vvvvvvvvvvvvo&endsec=vg&linechart=ELEC.PRICE.TX-ALL.M~ELEC.PRICE.TX-RES.M~ELEC.PRICE.TX-COM.M~ELEC.PRICE.TX-IND.M&columnchart=ELEC.PRICE.TX-ALL.M~ELEC.PRICE.TX-RES.M~ELEC.PRICE.TX-COM.M~ELEC.PRICE.TX-IND.M&map=ELEC.PRICE.US-ALL.M&freq=M&start=200101&end=202402&ctype=linechart<ype=pin&rtype=s&pin=&rse=0&maptype=0.
[13] U.S. Energy Information Administration. “Natural Gas Prices.” Accessed September 17, 2024. https://www.eia.gov/dnav/ng/ng_sum_lsum_a_epg0_prs_dmcf_m.htm.
[14] U.S. Energy Information Administration. “Electricity Data Browser.” Accessed September 17, 2024. https://www.eia.gov/electricity/data/browser/#/topic/7?agg=0,1&geo=vvvvvvvvvvvvo&endsec=vg&linechart=ELEC.PRICE.TX-ALL.M~ELEC.PRICE.TX-RES.M~ELEC.PRICE.TX-COM.M~ELEC.PRICE.TX-IND.M&columnchart=ELEC.PRICE.TX-ALL.M~ELEC.PRICE.TX-RES.M~ELEC.PRICE.TX-COM.M~ELEC.PRICE.TX-IND.M&map=ELEC.PRICE.US-ALL.M&freq=M&start=200101&end=202402&ctype=linechart<ype=pin&rtype=s&pin=&rse=0&maptype=0.
[15] Direct e-mail correspondence with Kent Marsh, Vice President for Capital Planning Sustainability & Campus Operations at Colorado Mesa University. June 27, 2024.
[16] U.S. Energy Information Administration. “What Is U.S. Electricity Generation by Energy Source?” Frequently Asked Questions (FAQs) – U.S. Energy Information Administration (EIA). 2024. https://www.eia.gov/tools/faqs/faq.php?id=427&t=3.
[17] U.S. Department of Energy. “Renewable Energy.” Office of Energy Efficiency & Renewable Energy. n.d. Accessed September 9, 2024. https://www.energy.gov/eere/renewable-energy.
[18] Building Decarbonization Coalition. “Zero Emission Building Ordinances.” 2024. https://buildingdecarb.org/zeb-ordinances.
[19] AHRI (Air-Conditioning, Heating and Refrigeration Institute). “Monthly Shipments.” AHRI. 2024. Accessed July 8, 2024. https://www.ahrinet.org/analytics/statistics/monthly-shipments.
[20] Jenkins, Jesse D. “What ‘Electrify Everything’ Actually Looks Like.” Mother Jones, 2023. https://www.motherjones.com/environment/2023/04/electrify-everything-scope-data/.
[21] Jenkins, Jesse D. “What ‘Electrify Everything’ Actually Looks Like.” Mother Jones, 2023. https://www.motherjones.com/environment/2023/04/electrify-everything-scope-data/.
[22] “About 70% of U.S. building stock was determined to be the amount that could be retrofitted with GHPs. So, it was 100% of the buildings that could use GHPs, which works out to about 70% of all buildings.” See: Liu, Xiaobing, Jonathan Ho, Jeff Winick, Sean Porse, Jamie Lian, Xiaofei Wang, Weijia Liu, Mini Malhotra, Yanfei Li, and Jyothis Anand. Grid Cost and Total Emissions Reductions Through Mass Deployment of Geothermal Heat Pumps for Building Heating and Cooling Electrification in the United States. No. ORNL/TM-2023/2966. Oak Ridge National Laboratory (ORNL), Oak Ridge, TN (United States), 2023. https://www.ecfr.gov/current/title-10/part-430/section-430.32#p-430.32(e)(1)(ii).
[23] Liu, Xiaobing, Jonathan Ho, Jeff Winick, Sean Porse, Jamie Lian, Xiaofei Wang, Weijia Liu, Mini Malhotra, Yanfei Li, and Jyothis Anand. Grid Cost and Total Emissions Reductions Through Mass Deployment of Geothermal Heat Pumps for Building Heating and Cooling Electrification in the United States. No. ORNL/TM-2023/2966. Oak Ridge National Laboratory (ORNL), Oak Ridge, TN (United States), 2023. https://www.ecfr.gov/current/title-10/part-430/section-430.32#p-430.32(e)(1)(ii).
[24] This assumes a 5% discount rate on the present value of currency.
[25] Buonocore, Jonathan J., Parichehr Salimifard, Zeyneb Magavi, and Joseph G. Allen. “Inefficient Building Electrification Will Require Massive Buildout of Renewable Energy and Seasonal Energy Storage.” Scientific Reports 12, no. 1 (2022): 11931.
[26] U.S. Energy Information Administration. “Commercial Buildings Energy Consumption Survey (CBECS).” Accessed September 17, 2024. https://www.eia.gov/consumption/commercial/.
[27] U.S. Energy Information Administration. “Commercial Buildings Energy Consumption Survey (CBECS).” Accessed September 17, 2024. https://eia.gov/consumption/commercial/.
[28] Buonocore, Jonathan J., Parichehr Salimifard, Zeyneb Magavi, and Joseph G. Allen. “Inefficient Building Electrification Will Require Massive Buildout of Renewable Energy and Seasonal Energy Storage.” Scientific Reports 12, no. 1 (2022): 11931.
[29] Buonocore, Jonathan J., Parichehr Salimifard, Zeyneb Magavi, and Joseph G. Allen. “Inefficient Building Electrification Will Require Massive Buildout of Renewable Energy and Seasonal Energy Storage.” Scientific Reports 12, no. 1 (2022): 11931.
[30] Feinstein, Laura, and Eric de Place. “Playing Monopoly, or How Utilities Make Money.” Sightline Institute, May 18, 2020. https://www.sightline.org/2020/05/18/playing-monopoly-or-how-utilities-make-money/.
[31] Seavey, Dorie. 2024. Leaked & Combusted: Strategies for reducing the hidden costs of methane emissions & transitioning off gas. N.p.: HEET. https://assets-global.website-files.com/649aeb5aaa8188e00cea66bb/663a27270c0fa4fffcfe447d_Leaked-and-Combusted-May-2024.pdf.
[32] Building Decarbonization Coalition. “A legislative heatwave: thermal energy network legislation updates (June 2024).” Accessed September 17, 2024. https://buildingdecarb.org/tens-legislation-june-2024.
[33] Bagdanov, George, Kristin Rider, Amy, and Claire Halbrook. “Neighborhood Scale: The Future of Building Decarbonization.” Building Decarbonization Coalition and Gridworks, November 2023. https://buildingdecarb.org/resource/neighborhoodscale.
[34] HEET. “Kickstart Massachusetts.” Accessed September 17, 2024. https://www.heet.org/Kickstart-Massachusetts.
[35] Vermont Community Thermal Networks. “How to Develop a Thermal Energy Network.” Accessed September 17, 2024. https://www.vctn.org/toolkit.
[36] Building Decarbonization Coalition. “A legislative heatwave: thermal energy network legislation updates (June 2024).” Accessed September 17, 2024. https://buildingdecarb.org/tens-legislation-june-2024.
[37] Bagdanov, George, and Kristin Rider. “Decarbonizing the Obligation to Serve.” Building Decarbonization Coalition, March 2024. https://buildingdecarb.org/wp-content/uploads/FINAL_Decarbonizing-the-Obligation-to-Serve_March2024.pdf.